LIQUID PHASE CARBON SYSTEM
General
Liquid phase granular activated carbon adsorption (GACA) is an effective and reliable treatment method. It is considered a best available control technology (BACT) by the USEPA and is a benchmark for other remediation technologies. In order for carbon adsorption to work well, it is important that the final design incorporate both the physical and adsorption process.
Minimum Carbon Bed Mass
The carbon bed must have sufficient mass to remove the contaminants in the water stream. This is a function of many factors, most of important of which is the mass rate of the contaminants (i.e. concentration and flow rate). Themass rate of contaminants may be calculated from Equation 1 as shown:
Breakthrough is defined as the point when contaminants are detected in the effluent and generally occurs when the adsorption capacity of the carbon is approached. Equilibrium saturation, or the maximum usable life of a carbon bed, may be determined from the following:
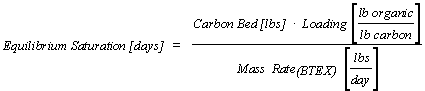 |
A well-designed GACA vessel should have sufficient contaminant mass removal capacity to allow for continued operation for a significant period of time before a carbon bed replacement is required. Most systems are designed to operate for a minimum of three months between carbon changes. However, several factors must be considered when determining the minimum carbon bed mass such as the travel time to and from the site, the operation and maintenance budget, space constraints on the site and within the equipment shed, the negative consequences of a discharge violation, etc.
Loading is a function of many variables including pore size distribution of the carbon, the molecular size and concentration of the contaminants, flow rate, compound solubility in water, and the presence of functional groups such as chlorine substitutions or aromatic structures. The performance of carbon is predicted by the adsorption isotherm. The isotherm is a measure of the amount of soluble organic that is adsorbed per unit weight of carbon at varying concentrations at constant temperature. The data collected from batch tests may be plotted on a log-log graph to form a straight line based on the empirical Freundlich equation:
 |
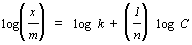 |
where,
x = amount of contaminant adsorbed (mg)
m = weight of carbon (g)
c = equilibrium concentration in solution after desorption (mg/l)
k = constant
n = constant
Isotherms are available for pure compounds, but these do not take into consideration the dynamics of adsorption when multiple compounds are present. As a result, carbon adsorption modeling is not reliable. However, for the purposes of comparison, pure compound isotherms may be used to calculate the total loading for a system.
After determining the mass of carbon required for the system operating parameters, the volume of the GACA vessel may be calculated. The amount of granular activated carbon required to fill a column may be determined as follows:
where AD is the apparent density of the carbon. The above equations may be combined to determine the necessary vessel geometry to allow continued operation of the system before breakthrough as shown in the following equation:
 |
Vessel Flow Configuration
Several adsorption vessel configurations are available including downflow, upflow, fixed-bed, fluidized-bed, pressure, and gravity. The downflow, fixed-bed adsorber is the simplest and most widely utilized design for groundwater treatment applications. The water enters the top of the vessel, is equally distributed across the carbon bed by the packed, flooded bed design, and is collected by slotted screens across the bottom of the vessel. The pressure type adsorber occupies less space and uses higher surface loading rates than gravity types. Nordic Water offers downflow, fixed-bed, pressure rated carbon adsorption vessels.
Hydraulic Loading
Hydraulic loading, or flux, is a measure of the volume of water flowing past a given cross-sectional area in a fixed amount of time. It is defined by the following equation:
Imagen7
If the hydraulic loading is too high, water will move too rapidly through the GACA vessel and, as a result, the adsorption efficiency will be reduced. Empirical data indicates that the hydraulic loading should not exceed 5 to 7 gpm/ft2. As an example, the maximum flow capacity of a 48-inch diameter GACA may be determined by rearranging the above equation and solving for the flow:
 |
 |
Velocity
The velocity is similar to the hydraulic loading; both terms are a measure of flux. To calculate velocity, the following equation may be used:
To convert hydraulic loading to velocity:
Using a maximum hydraulic loading of 7 gpm/ft2, the maximum velocity is calculated to be 0.668 ft/min.
Superficial Contact Time
Superficial contact time is defined as the volume occupied by the activated carbon divided by the water flow rate. It may be calculated from the following equation:
For a cylindrical GACA vessel, the above equation may be reduced to the following:
 |
The actual contact time is difficult to calculate, but is approximately one-half the superficial. Generally a superficial contact time of 10 to 15 minutes is adequate for treatment of contaminated groundwater. Longer times, however, may be required as the types of organic compounds in groundwater increase in number and concentration.
For short contact times (i.e. several minutes or less) the volume of water treated between carbon replacement increases sharply with increasing EBCT as the mass transfer zone occupies smaller and smaller percentages of the total carbon bed. The carbon usage rate may therefore be reduced by increasing the contact time of the vessel. The incremental changes in bed volumes processed decreases as the contact time increases, so that eventually further increases in contact time yield only marginal increases in the bed volumes processed. For TOC and trihalomethanes the frequency of carbon replacement increases dramatically as the contact time decreases.
GACA Vessel Geometry
In addition to flux and contact time considerations, the vessel geometry must be considered when designing a GACA system. The contaminant front moves through the GACA vessel in a plug flow manner. The shape of the plug is determined by many factors some of which include the type and number of contaminants, the flow rate, the adsorption rate, and the vessel geometry. If the shape of the vessel negatively alters the shape of the contaminant front, breakthrough may occur sooner than estimated. An API study determined that the optimum carbon bed height (i.e. GACA vessel height) is six feet. Using six feet as the minimum carbon bed height, 15 minutes as the minimum superficial contact time and the equations described above, the minimum cross-sectional area for a given set of conditions may be calculated from the following:
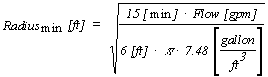 |
Using the example of a 48-inch diameter GACA vessel and its maximum flow rate of 63 gpm, this equation indicates that, for a single GACA, the minimum vessel must be 63-inches in diameter. In order to minimize the size of the vessel while still maintaining the minimum contact time, two GACAs may be piped in series. This will double the contact time while keeping the vessel size to a minimum.
Head Loss
Hydraulic head loss is related directly to the flow rate and inversely to the average size of the carbon particles. This may be seen in the following equation:
where,
The particle size also has a significant effect on the kinetics of the adsorption process where rates of adsorption increase with a decrease in particle size. A balance must, therefore, be reached between the optimum particle size for maximum adsorption versus minimum head loss. Commercially available particle sizes include 8 X 16, 8 X 30, 10 X 30, 12 X 40, 14 X 40, and 20 X 40, with effective sizes ranging from 0.55 mm to 1.35 mm. ARCE Systems recommends 8 X 30 mesh carbon for most groundwater treatment applications. This grade provides a reasonable compromise between good hydraulic characteristics and higher adsorption rates. This should be evaluated for sites with unusual conditions such as high TOC, low flow rates, compounds other than BTEX, or the presence of poorly adsorbed compounds.
|